Introduction
The ABB VFD (Variable Frequency Drive) ACS510 series is widely utilized in industrial applications due to its high efficiency, reliability, and ease of maintenance. However, users may encounter various fault alarms during operation, with F0035 (Fault 35) being a relatively common one. This article will combine the content of the ABB VFD ACS510 series user manual with relevant online information to provide a detailed analysis of the causes of F0035 faults and corresponding troubleshooting methods.
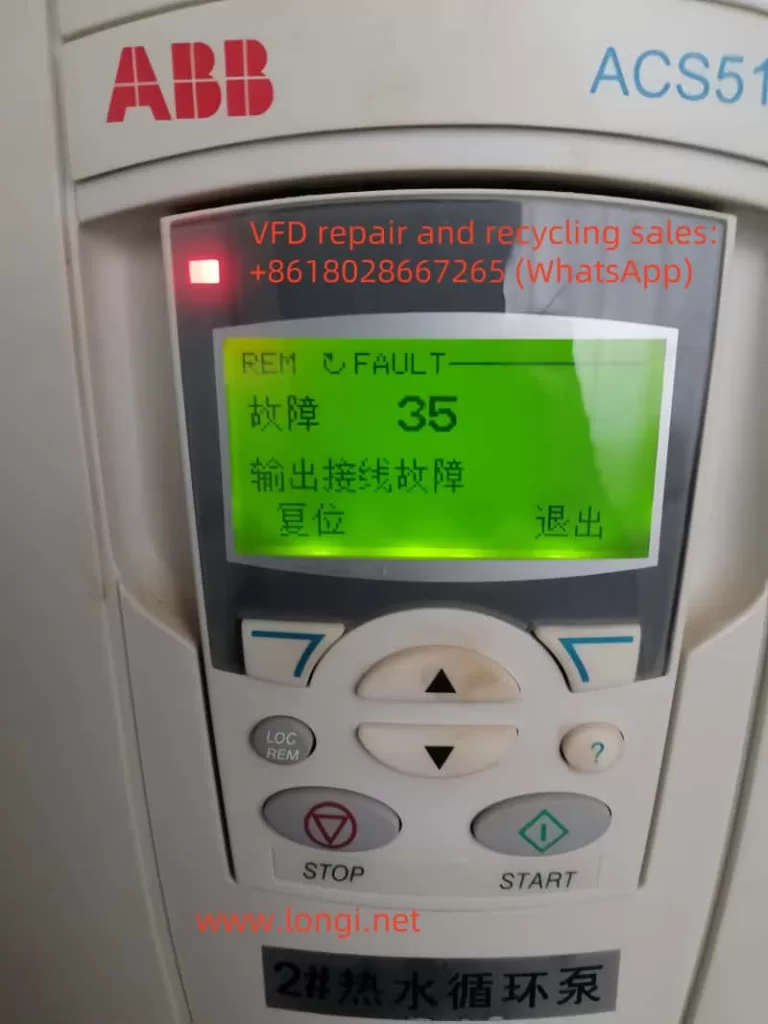
Overview of F0035 Fault
The F0035 fault, also known as “OUTPUT WIRING” fault, refers to an alarm triggered by the VFD when it detects incorrect connections between the input power cables and output power cables. According to the ABB VFD ACS510 series user manual, when the drive is stopped, this fault code monitors the correct connection of the input and output power cables. If a connection error is detected, the VFD will alarm and stop working to prevent possible equipment damage or safety accidents.
Cause Analysis of F0035 Fault
1. Incorrect Input Cable Connection
Incorrect input cable connection is one of the main causes of F0035 faults. If the supply voltage is mistakenly connected to the drive output terminal, the VFD will be unable to function correctly and will trigger an F0035 fault alarm. This connection error may result from negligence or misoperation by the wiring personnel.
2. Incorrect Output Cable Connection
In addition to incorrect input cable connections, incorrect output cable connections can also lead to F0035 faults. If the output power cables of the drive are connected improperly, such as reversed phase sequence or phase loss, the VFD will be unable to control the motor correctly, thereby triggering a fault alarm.
3. Capacitance Effect of Input Power Cables
In some cases, even if the input power cables are connected correctly, a large capacitance of the cables may cause false F0035 fault alarms. Especially when the input power cables are connected in a delta configuration, the capacitance effect may be more pronounced. This is because capacitance generates current in AC circuits, interfering with the normal operation of the VFD.
4. Environmental Interference
Environmental factors, such as electromagnetic interference, excessive temperature, and high humidity, may also affect the normal operation of the VFD, triggering F0035 faults. Particularly in industrial settings, electromagnetic interference is a non-negligible issue.
Troubleshooting Methods for F0035 Fault
1. Check and Correct Cable Connections
First, it is necessary to carefully inspect the connections of the input power cables and output power cables. Ensure that the supply voltage is correctly connected to the input terminal of the VFD, and the output power cables are correctly connected to the motor terminal, with phase sequence, phase, and other parameters meeting requirements. If any connection errors are found, they should be corrected immediately.
2. Disable Wiring Fault Detection Using Parameter 3023
If the capacitance of the input power cables is large and frequently triggers false F0035 fault alarms, consider disabling the wiring fault detection function using parameter 3023 WIRING FAULT. In the stopped state of the VFD, set the value of parameter 3023 to 1 to disable wiring fault detection. However, it should be noted that disabling this function may reduce the fault protection capability of the VFD, so it should be used cautiously.
3. Enhance Electromagnetic Interference Protection
For F0035 faults caused by electromagnetic interference, the following measures can be taken for protection:
- Use shielded cables or twisted pairs with better anti-interference performance;
- Install filters or isolation transformers at the input and output terminals of the VFD;
- Install the VFD away from sources of electromagnetic interference, such as high-power motors and high-frequency welding equipment.
4. Improve Operating Environment
To address F0035 faults caused by environmental factors, the following measures can be taken to improve the operating environment:
- Maintain cleanliness and dryness in the VFD operating environment to avoid the impact of dust and moisture on the VFD;
- Enhance ventilation and heat dissipation to ensure that the VFD operating temperature remains within the normal range;
- For VFDs installed outdoors or in harsh environments, add protective covers or take other protective measures.
5. Regular Maintenance and Inspection
Regular maintenance and inspection of the VFD are effective measures to prevent F0035 faults. Maintenance personnel should regularly check cable connections, measure input and output voltages and currents to ensure their normalcy, and clean dust inside the VFD. Additionally, they should pay attention to the operating status and alarm records of the VFD to promptly identify and address potential issues.
Conclusion
The F0035 fault is a common fault alarm in the ABB VFD ACS510 series, with causes including incorrect input cable connections, incorrect output cable connections, capacitance effects of input power cables, and environmental interference. To address these causes, corresponding troubleshooting methods can be adopted, such as checking and correcting cable connections, disabling wiring fault detection using parameter 3023, enhancing electromagnetic interference protection, improving the operating environment, and regular maintenance and inspection. By implementing these measures, the incidence of F0035 faults can be effectively reduced, improving the operational reliability and stability of the VFD.